The locked drawer, the unopened box – we all know how it feels to want to see inside. In most of us, curiosity peaks at childhood, or morphs, in adulthood, into a hobby. But there are those for whom the desire to see becomes an almost spiritual imperative, one that they must live by. They are scientists, in particular naturalists, and their impulse manifests in different ways. Some have looked outside the physical self, at the world around them or in the skies, while others have turned their attention inward, to the human body itself. It is a radical, disturbing about-face, a transgression across barriers, literally visceral, penetrating the membrane of our own skin. The story of anatomy must be told in images.
A relentless desire to reveal images once ran counter to the laws of society. The ancient Greeks viewed the body not as a divine vessel but rather as a source of pollution, and their sacred laws, etched into the stone walls of their sanctuaries, prohibited tampering with corpses. Skin, a symbol of wholeness, was inviolable. Mourners would bring out a corpse before dawn, when the streets were empty, to keep it from tainting passersby. The only sanctioned cutting of skin took place during animal sacrifice, but the skin itself was not burned, rather kept as a symbol of integrity. In the 4th century BCE, Aristotle – better-known for his philosophy than for his anatomical work, which was nonetheless invaluable – called for the practice of human dissection to investigate sources of pain and disease. ‘Even though a dead person also has the external form and shape,’ Aristotle wrote, ‘it nevertheless is not a human being.’ A bronze hand is not a hand, he argued, because it cannot perform its function. His redefinition of what is and is not human cleared the field for the first real examinations of the body.
Human dissection was only allowed during a brief window in antiquity. At the Museum in Alexandria – the metropolis that the Macedonian king Alexander the Great, Aristotle’s pupil, built in Egypt and named after himself – thinkers from Syria, Palestine, Crete, Cyprus, Ionia and Mesopotamia studied and exchanged ideas, enriching the fields of astronomy, philosophy, zoology, mathematics and medicine. Like Aristotle, philosophers in Alexandria considered dead bodies to be nothing more than inert objects, and so the superstitious prohibition did not apply. For the first time on record, the Ptolemaic kings of Alexandria allowed two Greek physicians, named Herophilus and Erisastratos, to dissect the dead and cut or surgically repair and explore (vivisect) the living, observing ‘parts which beforehand nature had concealed’. The noun ‘anatomy’ comes from the ancient Greek word temnein – meaning ‘to cut’. Each cut revealed another unknown region.
When Theodosius destroyed Alexandria in 389 CE, after Julius Caesar had burned part of the library in 48 BCE, the practice of human dissection came to an end. The Church, spreading Christianity during the Middle Ages, prohibited dissection, which it considered blasphemous. Dissection went out of practice until 1315. Then, in the 16th century, the Belgian physician Andreas Vesalius performed his own masterful dissections. The result was his magnum opus On the Fabric of the Human Body (1543), the most consequential work of anatomy ever written, not only for its words but also for its illustrations, more than 200 breathtaking woodcuts executed on pear tree boards by a group of artists who may have included a disciple of the Renaissance master Titian. Human bodies, stripped of skin, were posed expressively and rendered in exquisite detail, like portraiture subjects. It was Vesalius who showed that there is no physical outlet through the skull, that the brain is bathed alone in its bone casing. Visual imagery was now indispensable to the study of anatomy, with images becoming aesthetic objects in their own right.
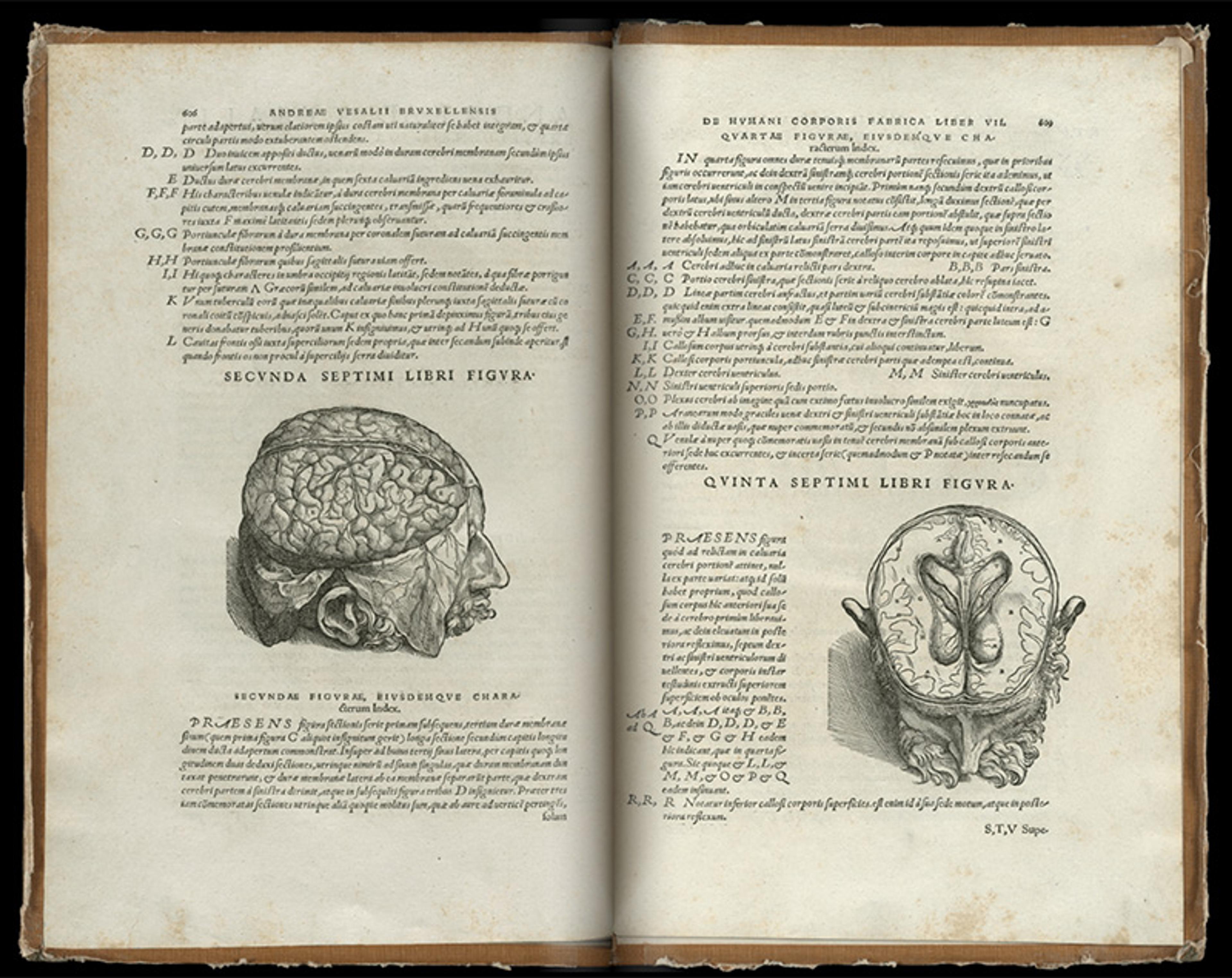
No one merged art and science quite like Leonardo da Vinci, who trained himself to draw human forms by studying anatomy. By the end of his life, he claimed to have dissected 30 corpses. ‘You may perhaps be deterred,’ he wrote in his diaries, ‘by the fear of passing the night hours in the company of these corpses, quartered and flayed, and horrible to behold.’ His anatomical contribution consists of 18 double-sided pages of drawings, more than 240 of them, with more than 13,000 words of notes. Now, the unseen was not only seen – it was beautiful.
Fleas become as large as lambs, Galileo said, so a word for early microscopes was ‘flea glasses’
Glimpses into our anatomy drove curious minds to see more. Toward the end of the 16th century, a revolution in seeing occurred when, according to legend, two children at a lensmaker’s shop in the Dutch Republic held two lenses against each other and saw the nearby church looming larger and closer. Town officials sent a letter to The Hague heralding the discovery of a ‘magical device’.
As Galileo noted, these lenses could also be used ‘for observing at close quarters the smallest objects’. He called this microscope the occhialino or ‘little eye’. Fleas become as large as lambs, Galileo said, and so a word for early microscopes was ‘flea glasses’.
At first, people treated the instrument as nothing more than a novelty item, a toy for wealthy children hawked by spectacle makers and travelling salesmen. In the evenings, well-to-do families would gather in the parlour and look through the microscope for entertainment. No one seemed to understand the transformative power of the novel lens. The aesthetics of the images were stimulating, but there was no appreciation of their scientific meaning.
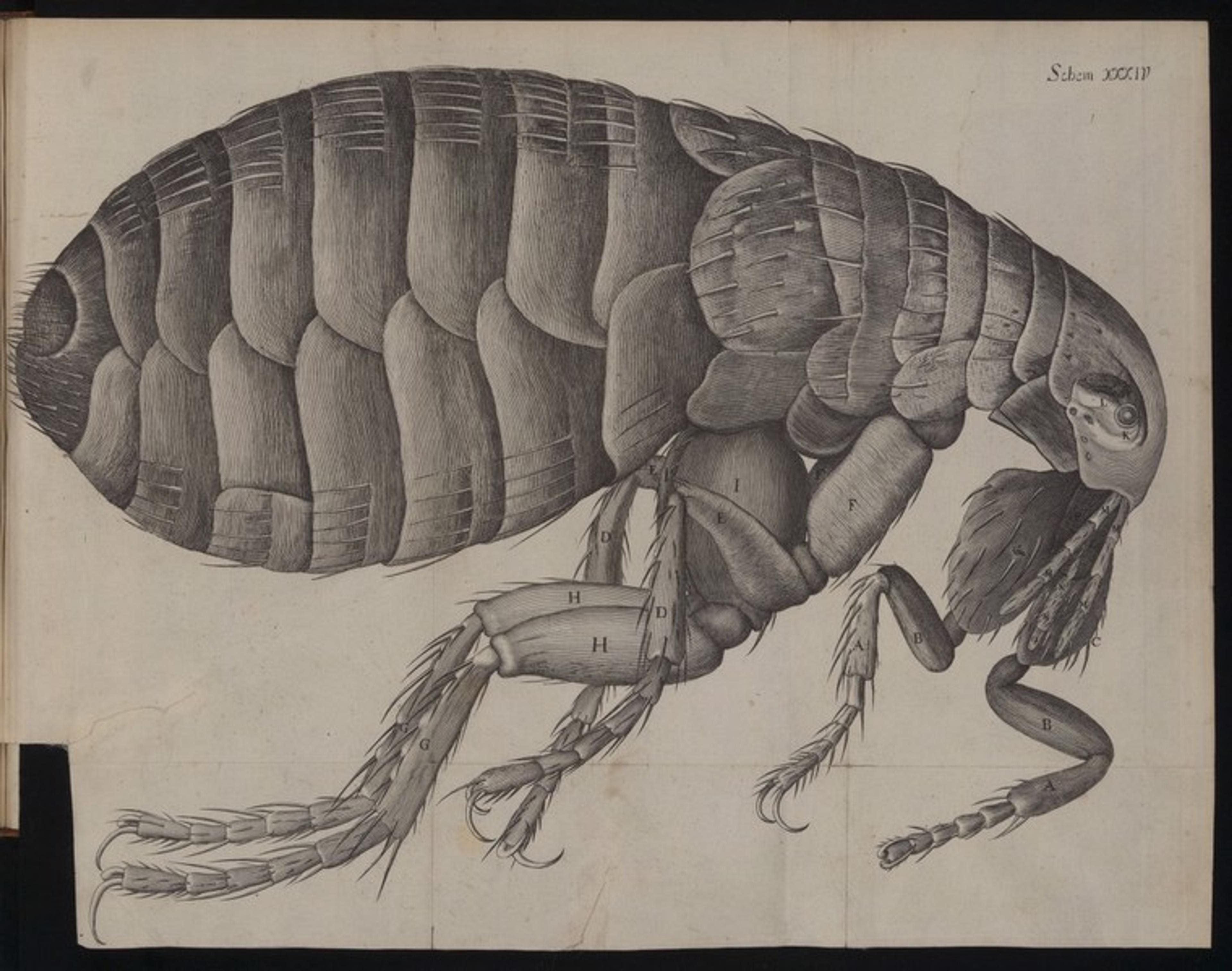
The British naturalist Robert Hooke helped scientists make sense of what they were seeing when he published the first scientific treatise on microscopy – Micrographia (1665) – which became a bestseller. The illustrations, once unfolded, spanned multiple pages. ‘By the help of Microscopes,’ Hooke said, ‘there is nothing so small, as to escape our inquiry.’ When he examined a thin sliver of cork under the microscope, he noticed what looked like a sequence of empty boxes, which reminded him of the living quarters of monks – called cellula – from the Latin meaning ‘little rooms’, and so the microscopic structures became cells. His famous image of cells, like two spread-out swatches of porous fabric, signifies a whole new realm of life sciences.
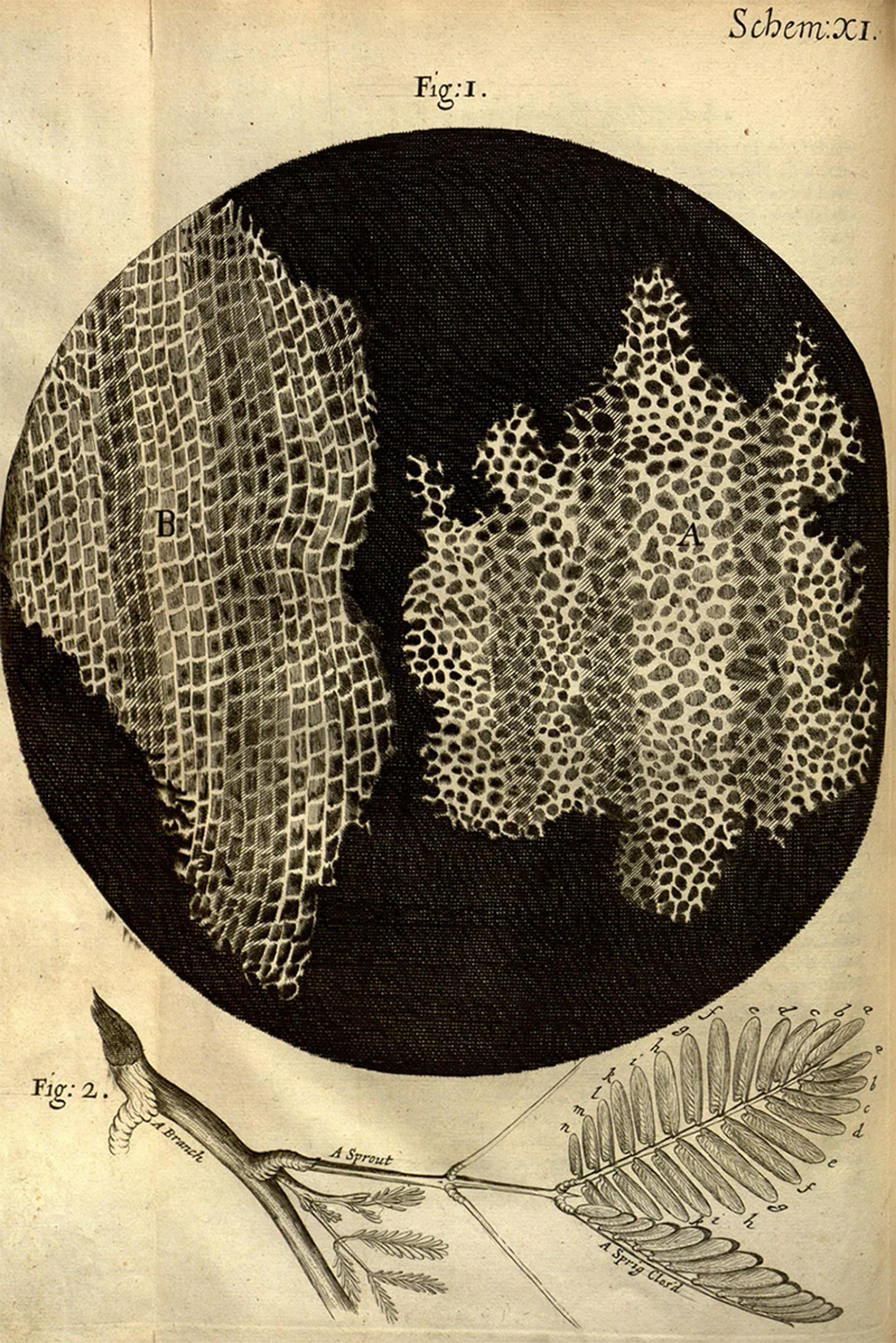
Scientists would not formulate cell theory itself for another almost 175 years but, if not for microscopes, we would have never discovered the world of the infinitely small, including not only cells but also bacteria, which, two centuries later, allowed researchers to devise treatments for previously unknowable diseases.
By the turn of the 19th century, the French anatomist Xavier Bichat had emphasised yet another level of anatomical organisation when he introduced the word tissue, the past participle of the verb tisser, meaning ‘having been woven’. Organs proved to be complex entities, and so, deftly with his scalpel, he teased apart their fabrics, which he declared the smallest elements of the body. (Cloth, wool, lace, warp and woof, spinning, thread, and embroidery all show up as metaphors in old anatomical treatises.) Bichat refused microscopes, which he deemed untrustworthy, instead relying on his naked eye, which nonetheless led him to accurately identify 21 kinds of tissues, an amazing feat considering how he handicapped himself.
In 1819, the German anatomist Karl Mayer coined the term ‘histology’, joining the words histos (tissue) and logos (study). With histology, the microscopic study of tissue, the possibility of a new set of images, and a new level of organisation, dawned. Back in 1661, the Italian physician Marcello Malpighi, perhaps the first histologist to attempt a microscopic investigation of the nervous system, had discovered capillaries by injecting black ink into pulmonary arteries, which later contributed to William Harvey’s theory of circulation, allowing modern doctors to understand and treat heart disease.
The dream of histologists was an impossible one. What they longed for was a kind of resurrection, to encounter dead tissue as though it were still alive. However, as soon as an organism perishes, its body starts to decompose. To maintain the organic structure of tissue, the histologist has to halt the natural process of decay, since, with every passing moment, its structure further degrades until every cell is broken down completely. Preservation is a feint towards immortality, and human beings have tried to preserve life since ancient times. The ancient Egyptians mummified bodies with natron, a salt mixture harvested from dry lake beds, and stored organs in canopic jars, the lids of which were usually carved with heads of the sons of Horus for protection. The process of preserving tissue, which became known as fixation, was similar to that of developing a photograph, rendering the paper insensitive to further action by light. Just like photography, histology yielded images of the world that could not only be recorded but also shared.
When he washed the flask with ethanol, he saw a brilliant purple colour
Cells alone are slippery to image; being mostly water, they appear transparent under a microscope. But, like fabric, they absorb certain dyes. In 1673, Antonie van Leeuwenhoek, a contemporary of Hooke’s, who encountered lenses as an apprentice to a draper and examined myriad substances, including his own bodily secretions, applied dye extracted from the bulb of Saffron crocus, a large purple flower, to examine muscle fibre. Studying the histology of wood, in the following century, the British botanist John Hill relied on carmine, made from the dried and ground bodies of female cochineal parasites clustered on cacti in Central America, which the Aztec and Maya had discovered.
Carmine would prove to be an invaluable histological resource that made an aesthetic imprint on the field. In 1858, the German anatomist Joseph von Gerlach walked into his local drugstore and asked the apothecary to recommend a chemical agent with which he might be able to colour blood vessels, and the apothecary suggested the dye. Gerlach injected it into the bloodstream, but it slipped into the bordering tissue, and nearby cells picked it up. He tried to stain a section of cerebellum, an area of the brain, but he obtained bad results and went home dejected. It turns out that he accidentally left pieces of tissue in the solution, and the next morning the stains were spectacular. ‘To be an histologist,’ one historian of science later said, ‘became practically synonymous with being a dyer.’ The visual language of microscopic images now included colour.
Dyes had come from unexpected places. In the early 1700s, a paint manufacturer in Berlin, Johann Jacob Diesbach, was sharing the laboratory of Johann Konrad Dippel, an alchemist working to distil bone oil from animal blood mixed with potash, a salt. Diesbach tried to produce a red pigment by boiling cochineal, but he needed potash, and so he borrowed some from Dippel. Unbeknown to him, the substance was contaminated and meant for the trash, and so, instead of turning red, the mixture paled, and then, when Diesbach concentrated it, turned purple, and finally blue. At the time, there was no affordable or stable blue for dyers to use because, in those days, ultramarine, derived from lapis lazuli, came only from the mountains of Afghanistan and cost more than gold. ‘Prussian blue’, as the new pigment was called, was a 10th of the price of ultramarine, and it soon made its way to Paris, then around the world. Artists used Prussian blue for painting; it is the pigment in Vincent van Gogh’s The Starry Night (1889), and the Japanese imported it for woodblocks, most famously used in Hokusai’s The Great Wave off Kanagawa (1830-33). Prussian blue is related to prussic acid found in Zyklon B, the poisonous chemical that Nazis used in the gas chambers of their concentration camps.
If necessity is the mother of invention, then accident is its midwife. In 1856, an 18-year-old university student in London, William Henry Perkin, was experimenting in his home laboratory over Easter break. In an effort to impress his chemistry professor, he was trying to find a cheap way to synthesise quinine, a treatment for malaria, which is commonly made from the bark of a cinchona tree, native to South America. Perkin worked with aniline, a chemical found in coal tar, the oozy sediment left from Victorian-era gas lighting, which was thought to have a similar chemical structure to quinine. Surprisingly, what remained from his experiment was a black residue. When he washed the flask with ethanol, he saw a brilliant purple colour, which he successfully transferred onto a cloth.
It just so happened that purple was the most fashionable colour at that time, and previously had to be extracted from a kind of shellfish that lived only in the Mediterranean. Coal tar, on the other hand, was plentiful and, given the increasing number of gasworks in Britain and Europe, it was relied upon by a growing population for lighting, heating and cooking. The dye was not expensive, and the colour did not fade after washing, as others did. Perkin dubbed it ‘mauve’. When Empress Eugénie wore a silk mauve dress that summer, the fashionable ladies in Paris all imitated her. Magentas, yellows, blues and pinks were soon created. The German biologist Paul Ehrlich systematically studied these aniline dyes and forged relationships with representatives from the dyestuffs industry, expanding the histological palette. We tend to think of science as highly organised and planned but, in reality, it is often the story of earnest mistakes and those perspicacious enough to capitalise on them.
The histological process is temperamental and intricate. After fixation, samples must be dehydrated, cleared, and embedded in melted paraffin, then cooled to form a solid block and cut by hand or with an instrument called a microtome, then mounted on the glass slide, cleared of paraffin, and stained with dyes. Every fixation method had its drawbacks. Thinner slices might take minutes, while bulkier tissue required hours, and some fixatives worked over the course of months. If the tissue was dehydrated for too long, then it would shrink and become brittle. If it was hardened for too long, it would not absorb the dye. The sample was washed, eliminating any chemical residue but, if washed for too long or at too low a temperature, the tissue became too pale to be easily studied. Each chemical used in the process had to be miscible with the last, so that the proportion of the chemical added could be steadily increased until it replaced the preceding one. The tissue had to be cut so that the thickness of each section was uniform – otherwise the depth of the image would not be consistent, which would threaten the accuracy of any observations.
There is something alchemical about histology. But instead of transmuting base metals into gold, the histologist brings forth the hidden structures of cells, like a magic trick, in vivid colour.
The brain, because it is so densely packed with fibres, proved impossible to stain clearly
Technological advances in the 19th century considerably eased the process. In 1859, the Russian chemist Aleksandr Butlerov discovered the preservative formaldehyde for use as a fixative. In 1842, the German anatomist Benedikt Stilling had come upon another, non-chemical one when he left a section of spinal cord tissue on the windowsill in the -13ºC cold, and returned the next day to find it frozen solid. In the 1830s, the Czech anatomist Jan Evangelista Purkinje invented a precision microtome, a device for slicing tissue extremely finely, on the order of micrometres. Canada balsam, a semi-solid extract of resin and oil that appears as prominent blisters on the smooth, thin bark and branches of young balsam fir trees in North America, served to glue the stained tissue between the slide and glass coverslip. Perhaps most importantly, in the 1820s, the British amateur scientist Joseph Jackson Lister fashioned lenses that corrected for chromatic and spherical aberrations, distortions that obscured the microscopic image, allowing for the discovery of cells and bacteria.
A flood of new images arrived like never before. Aided by histology, 19th-century researchers revealed the tissues of the body: epithelia, muscle, bone, eye, benign and malignant tumours, and spinal cord. Histology textbooks appeared; medical schools started teaching the subject. By the 1870s, the only tissue that histologists had not seen clearly was that of the nervous system. The brain, because it is so densely packed with fibres, proved impossible to stain clearly. Scientists assumed that brain matter formed an impenetrable tangle of interconnected fibres, known as a reticulum. In 1873, working in the kitchen of a hospital outside of Milan, the histologist Camillo Golgi invented a new stain that affected only a small percentage of cells, effectively pruning back the thicket. He called it the black reaction, for its stark colour, which stood out like ink against parchment.
The apotheosis of histology, and perhaps the most spectacular revelation in the history of anatomy, came in 1888 when Santiago Ramón y Cajal, an unknown Spanish researcher, improved upon the black reaction to demonstrate the anatomical independence of nerve cells, later termed neurons and defined as the basic units of the brain. Cajal became the first person to use the term plasticity when referring to the central nervous system and its ability to change its structure in response to experience, introducing a concept that has become en vogue in many modern fields, including psychology and education.
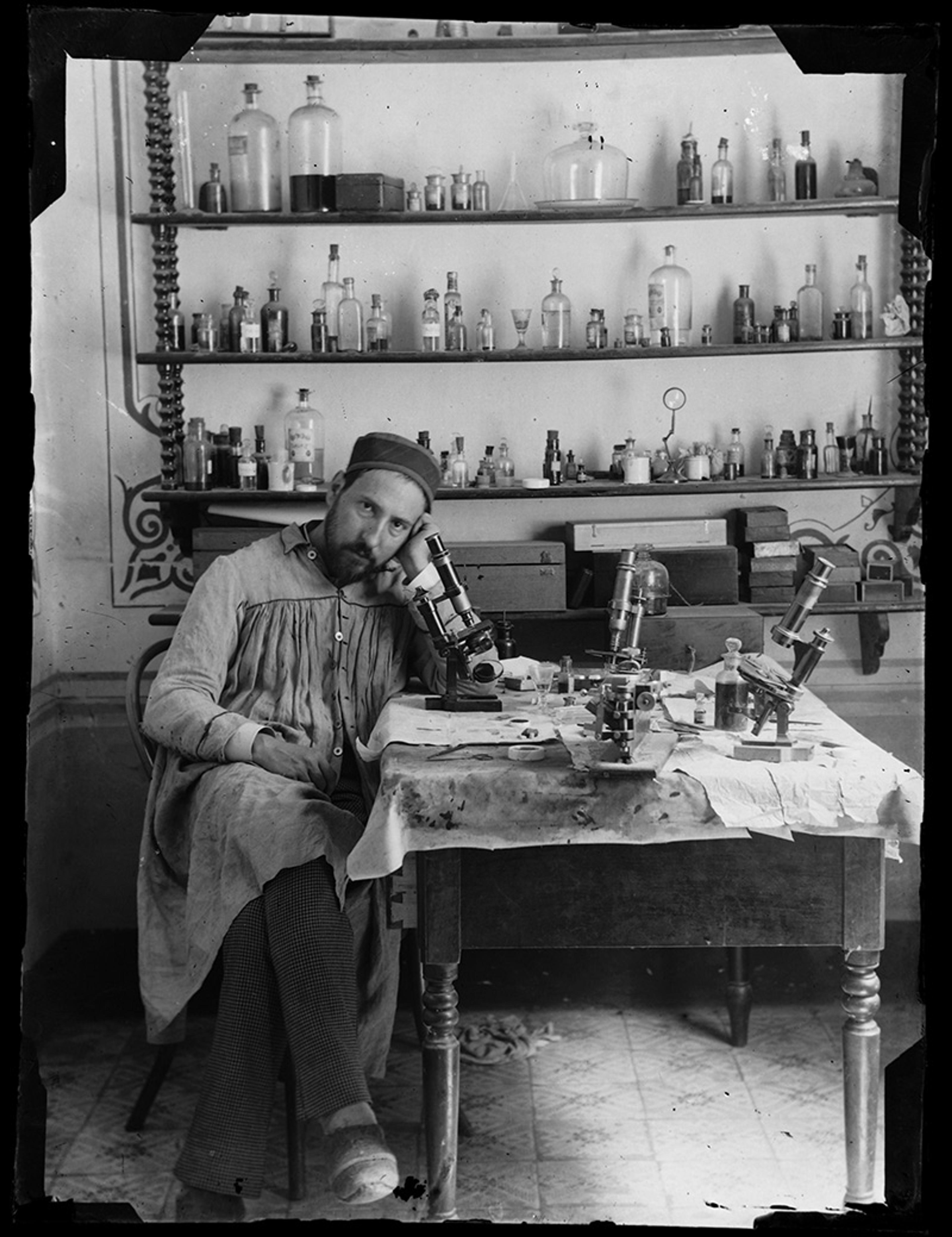
Cajal grew up in the mountains of northern Spain wanting to be an artist, despite the opposition of his tyrannical father, a physician who insisted his son study medicine. Before the advent of photomicroscopy, histologists had to draw their findings in order to disseminate them. Cajal was a superb draughtsman, and his images of neurons have become iconic. Their significance extends far beyond anatomy; since Hippocrates, many have recognised the brain as the enigmatic source of the mind. And so, to see Cajal’s neurons is to glimpse the hidden structures – in his view, the living beings – behind our whole experience of being alive.
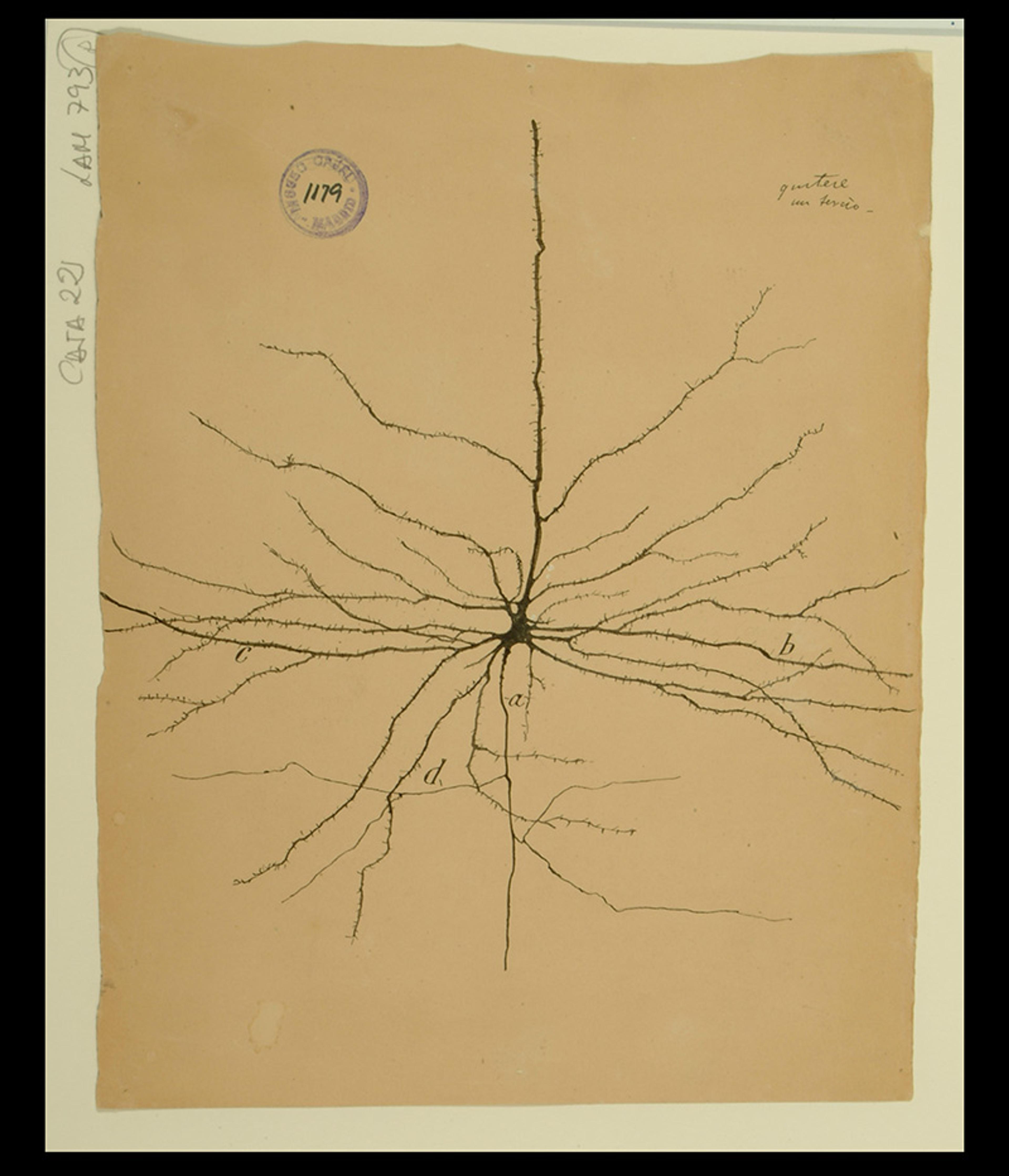
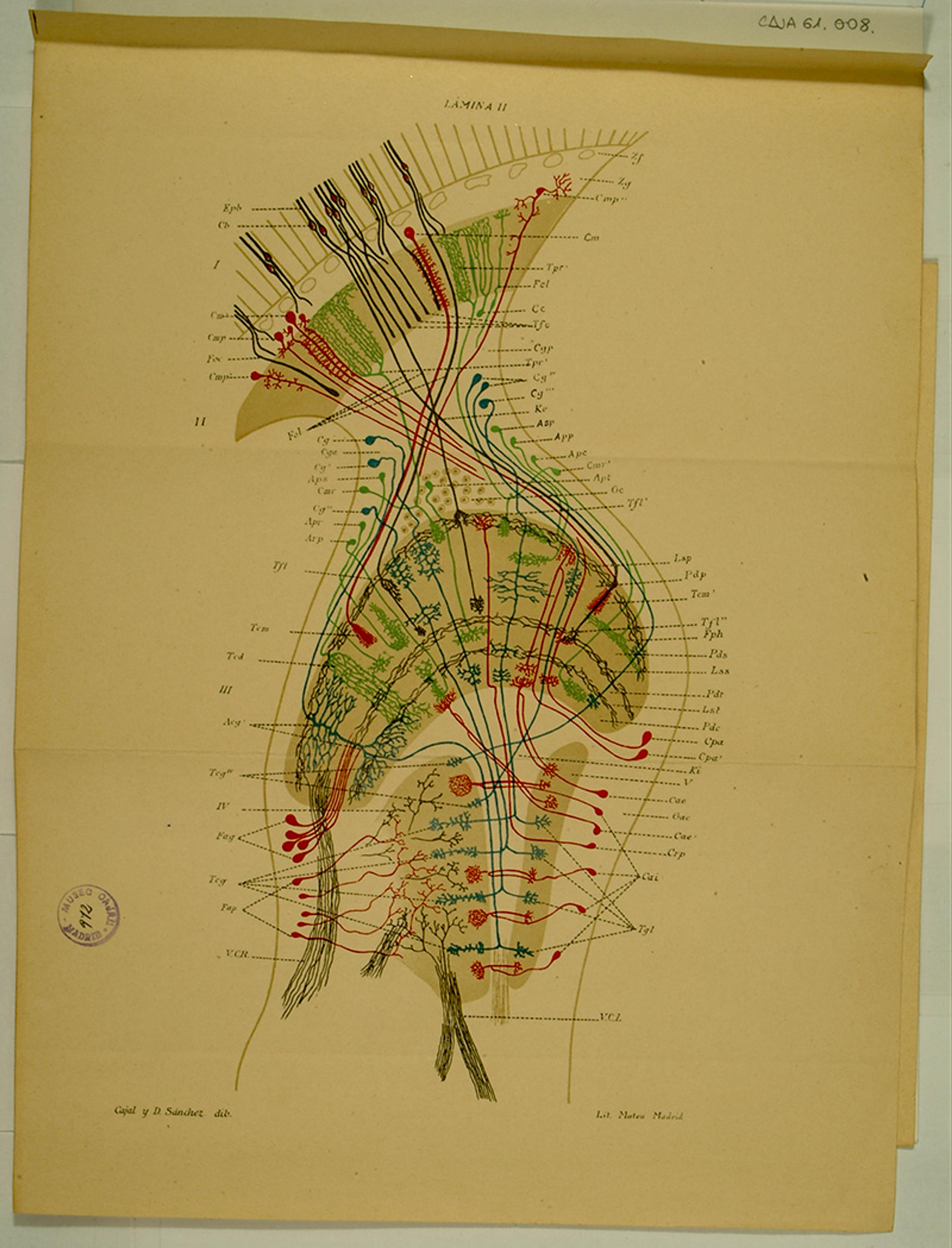
More than 100 years later, statistics and machine learning dominate neuroscience, presenting the brain in terms of data sets, abstracting flesh-and-blood into mathematics. The brain is often called the most complex object in the known universe, and that may be true, yet it is hard not to detect a certain egomania behind that statement. We want the brain to be the most complex because then, when we finally arrive at the nirvana of complete self-understanding that we implicitly expect, we will have realised something almost supernatural rather than mundane.
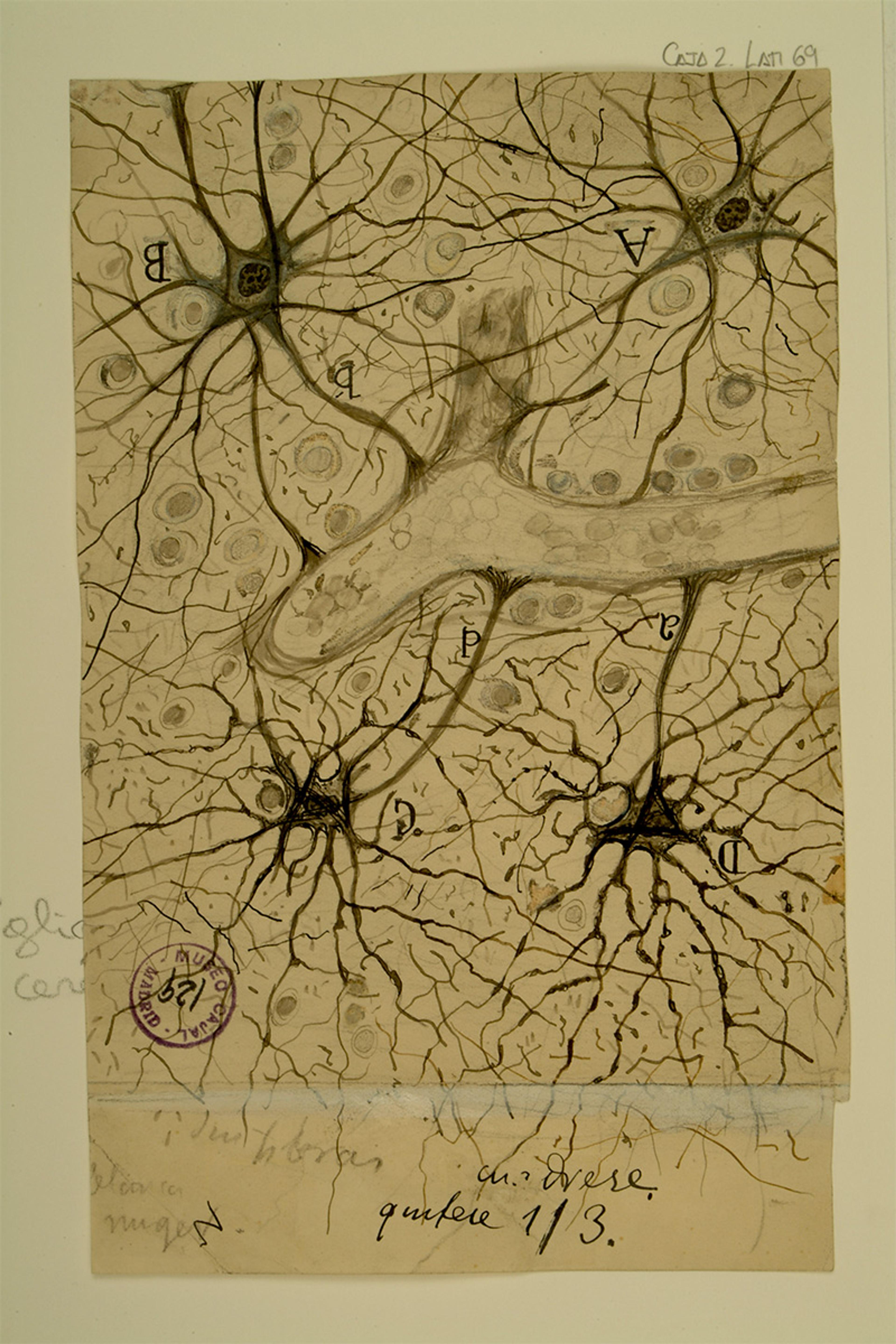
Seen from the outside, the brain is a wrinkled, grey lump; inside, as histology revealed, it is full of wonder. Has it been our secret hope for all these millennia to see the brain as beautiful, like the face of a god who loves us? The brain is far more than just another biological organ; its most recently evolved areas symbolise our humanity, distinguishing us from our fellow animals. The recursive puzzle of neuroscience, at once maddening and enticing, is that we externalise the brain, viewing it at once outside ourselves and inside us. In this way, neuroscience is the ultimate act of self-reflection – it is the brain that sees itself.
In this collage of vibrant colour, cell bodies appear like neon raindrops
Imaging technology in the 21st century is beyond what Cajal and his contemporaries could have imagined, but our desire remains the same. We have preserved the structure of our own enquiry. Gone may be the days of dusty, cork-stopped bottles filled with eerie fluids, of cheap light microscopes, of practising microscopy alone in the kitchen or the attic. Yet cells, no matter how you image them, are still mostly water. Adding colour is still necessary.
The histology of the 19th century has led directly to a world of unprecedented clarity and vividness, promising immaculate control of complex processes, no longer susceptible to the vagaries of laboratory chemistry. A technique called immunofluorescence, first described in 1942 and refined in 1950, employs antibodies tagged with fluorescent compounds that reemit light upon excitation and thus function, in the eyes of an observer, like dyes. Another technique, CLARITY (Clear Lipid-exchanged Acrylamide-hybridised Rigid Imaging/Immunostaining/In situ hybridisation-compatible Tissue-hYdrogel), renders tissue transparent with monomers called hydrogels, allowing immunofluorescent stains to expose neurons in the whole brain.
A technique known as optogenetics became popular at the start of the 21st century. Instead of chemical reagents, optogenetics relies on photosensitive proteins, or opsins, first discovered in algae; instead of revealing the structure of cells, the technique tracks their function. With genetic engineering, researchers add new code to certain neurons that allows them to make opsins. Then they shine a certain kind of light at those neurons to open their ion channels and fire the electrical signal, thus stimulating their activity. By precisely controlling the processes of the engineered neurons, researchers can observe how other neurons respond, thereby illuminating the brain’s circuitry, carefully and selectively. Already, optogenetics has been used to help patients recover after a stroke and to provide insight into the mechanisms behind anxiety. Scientists hope that their findings will lead to advances in treating Alzheimer’s and PTSD. But, technically speaking, optogenetics is a method of perturbing not imaging; the colours are useful only to turn neurons on and off.
The most obvious heir to the spirit of Cajal’s histology is a technique called ‘Brainbow’, which introduces coral, jellyfish and sea anemone DNA into mice, fruit flies and other small animals. The fluorescent proteins produced as a result are blue, cyan, yellow, orange and red. When mixed, they create a palette of hundreds of distinctive hues. In this collage of vibrant colour, cell bodies appear like neon raindrops. Unlike the black reaction, Brainbow inventors say, their technique can trace many neurons at once. Neuroscientists these days are far more concerned with populations of neurons than they are with individuals. Yet there is something intimate and revealing about the staining of a single cell, like the portrait of a human being. ‘Only true artists are attracted to science,’ Cajal said.
In researching my latest book, The Brain in Search of Itself (2022), I found myself in Cajal’s archives, located in Madrid at the institute that bears his name. Histological slides are not just images but objects in their own right, idiosyncratic and tangible. Cajal’s bore old labels with his name and university position, along with the designation ‘b’, meaning bueno (‘good’), all the way up to ‘b b b b b b’ for the absolute best. One can place them on a microscopic stage today and still see what he saw more than a century earlier. In surviving him, they have acquired new life.
Histological slides are like manuscripts written by hand; something exists therein about the person who made them. It is the beauty of humanity, our fallibility, our imperfection, our devotion to the impossible, if not the absurd. New techniques open up new possibilities for scientific discovery, but what have we lost? Something sensual, perhaps. The end of a kind of craftsmanship, of what is handmade, a loss of contact with the source material, which comes from our body, or the absence of smell. They are more primal than the drawings of them, one stroke further upstream, towards the source. Standing in the archive at the Cajal Institute in Madrid, the stained tissue on his slides, mounted with resin, still felt gummy to my touch.